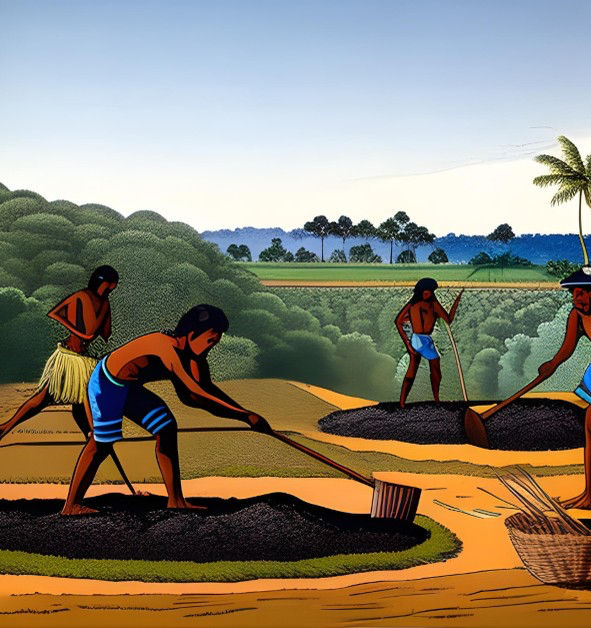
Soil. Society.
The Soil Society project explores how architecture and waste management systems can shift from pollutant to regenerative. Unlike sustainable design, which focuses on minimizing harm, regenerative design actively improves ecosystems. The system integrates aerobic composting, anaerobic digestion, and hydroponics to provide fresh food, warm water, and warmth while producing nutrient-rich humus soil as a byproduct.
Current agricultural and waste water treatment systems contribute to soil degradation, water pollution, and high energy consumption, mainly due to the reliance on synthetic nitrogen and energy intense wastewater treatment. This project breaks the carbon cycle by sequestering atmospheric carbo by turning it into and storing it as soil and closes the nitrogen cycle by returning nutrients, once taken by crops back to the soil. It transforms "waste" into valuable resources, helping to regenerate ecosystems and sequester carbon.
The Challenge
Modern wastewater treatment systems significantly degrade the environment by oxidizing nutrients in waste, turning them into gases like CO2, N2, and N2O (nitrous oxide) rather than returning nitrogen to the soil. This disrupts the natural nutrient cycle, depleting the soil and increasing reliance on synthetic nitrogen fertilizers.
Plants take carbon from the atmosphere, but they rely on nitrogen from the soil to grow. Current systems fail to recycle nitrogen, forcing agriculture to depend on synthetic fertilizer produced by the Haber-Bosch process,
which consumes 1-2% of global energy and produces 60% of N2O emissions—a greenhouse gas 289 times more potent than CO2.
This reliance depletes soil health while raising energy consumption
and emissions.
Water-based sanitation systems also waste drinking water and release pollutants into ecosystems, causing issues like algal blooms. Instead of treating human waste as a valuable nutrient for soil, it is discarded, further harming the environment.
To solve this, soil-based sanitation, which treats waste as nutrients for soil regeneration, reconnecting the nitrogen cycle, restoring soil health, and reducing dependence on synthetic fertilizers and energy-intensive processes, is a promising approach.
Feasibility Simulation:
To test this system and its thermodynamic requirements, I have simulated its metabolism using grasshopper and Python. Monitoring the systems thermodynamic energy requirements (30C for ideal anaerobic environment) and heat production of the compost. Since the compost is designed to surround the anaerobic digestion tank, it functions as a heat source and as an insulation.
The heating energy needs of anaerobic tanks are directly influenced by the thickness of the insulation (compost) surrounding the tank. These two factors are interdependent, with the ideal balance achieved by intersecting the energy demand graph (dependent on insulation thickness) with the energy production graph (in relation to compost volume/insulation thickness). It also depends on parameters such as the number of users, outside temperature, amount of food waste. As this influences the size of the system.
The simulation additionally tracks the C:N ratio at these intersection point. This ratio, ideally around 1:30, is crucial for optimal heat and hummus production. Depending on the user size, this intersection point can result in an excess of nitrogen in the system. The solution is to add more carbon to the aerobic compost to restore the balance.
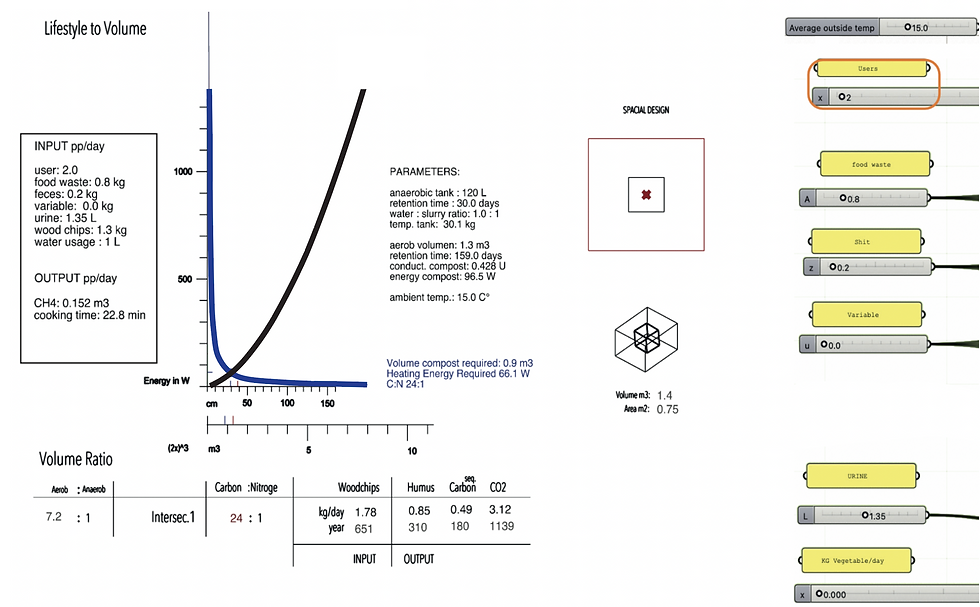
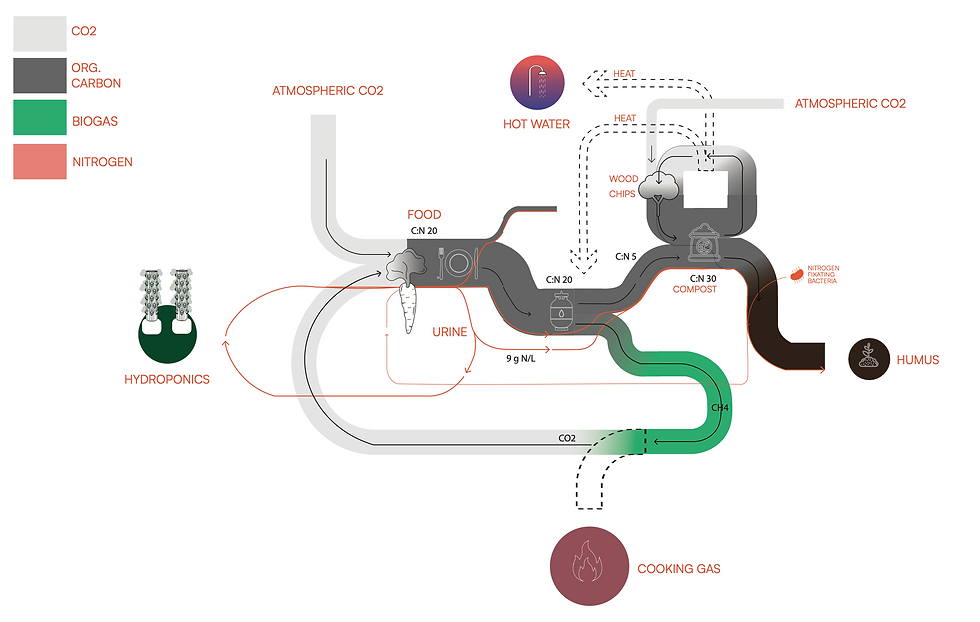
The increase in carbon means an increase in compost. This leads to additional heat production, which can be used to heat household water. The red graph can monitor this energy addition / warm water production.
Alternatively to adding carbon (wood chips or paper waste) to the system, nitrogen can also be removed to achieve the right balance. Some of the enriched nitrogen from the anaerobic process (slurry) can be redirected into a hydroponic system instead of added to the compost. This system will return the nitrogen directly to the crop - skipping the soil. In this way, warm water and Food production are negatively correlated, and users can use the script to find their ideal personal balance.

Design & Fabrication
I developed a prototype for a system designed to support two people. The scripted simulation informed the prototype.
The building process involved the use of widely available materials, ensuring its accessibility and reproducibility. The fabrication of this prototype allowed for real-world testing against the simulated results.
Once built, the system was monitored to compare the simulated predictions with the measured real-world performance. Notably, the actual system proved slightly more efficient than the simulation had predicted, particularly in its heat generation and energy recovery. Based on these observations, the script was adjusted to improve its accuracy for future iterations, incorporating data from real-world performance to fine-tune its predictive capabilities.
This iterative process of design, simulation, and testing ensured that the prototype validated the theoretical framework and provided actionable insights for scaling the system or applying it in different contexts.


City Model
The newly adjusted simulation for a 30-person urban scale system gave the following results:
-
30 liters of warm water per person per day.
-
180 grams of fresh vegetables per person per day.
-
26 minutes of cooking gas per person per day.